Introduction
Earthquake engineering is the study of how a structure performs when subjected to the forces of an earthquake. Because earthquake forces are more complex than other structural forces, physical and digital simulation methods are used to validate the seismic performance of a structure. The physical method of testing is done with the use of a shake table, and the virtual methods use computer model simulations using either static or dynamic methods of mathematical assessment (pseudo-static and pseudo-dynamic). These tests are performed on new materials, partial configurations, or full designs. Building codes often use the results of these tests when establishing design requirements for specific constructions. History has shown that buildings designed using earthquake engineering can save lives in seismically active zones. Earthquake simulations play an important role to protect the safety of the people that use or are in proximity of a structure. The following paragraphs provide an analysis of literature about shake table and pseudo-static/dynamic methods to assess the trends of how, when, and why specific methods are being used. The analysis of different tests aims to help determine which test would be the most useful in evaluating the seismic performance of earthbag construction.
Shake table testing
Shake table testing, is a physical dynamic test on which the structure is placed on a platform that moves on one or more axis. The shaking is meant to simulate an earthquake’s movement, and accurately represent the forces involved. This is the most widely recognized test and is often used to validate other models and analysis. Some codes require multi-axial testing on earthquake simulators (shake table testing) to qualify even non-building components, such as electrical substation equipment.1 The shake table test is the only way to study the performance of structural systems that are influenced by the dynamic and spatial characteristics of earthquake action, such as masonry. The test consists of a platform, a guiding system and actuators. The greatest challenge is to accurately represent the earthquake motion.2
The tested structure can be a full-scale model or sized down. The ratio of the model to prototype is generally determined according to the load capacity and dimension of the available shake table.3,4 There are tests with 1/3 scale 4 story buildings, structures that are scaled down to 1:6, and in the case of a massive structure such as a cooling tower, the model is scaled 1:30. If possible, full scale structures are also tested. Tests have also been done where modular brick construction is scaled by one half.5 It is important to note scaling removes mass that contributes to inertial forces. This loss must be made up with artificial mass and must be positioned carefully to make sure it does not affect other parameters of the structure.3 The shake table method permits the testing of irregular shaped buildings that may be more difficult to model digitally.6
A shake table with three axes (6 Degree-of-Freedom) is the most accurate, but some tests require less, and other studies do not have the possibility of a shake table with three dimensions of movement and so researchers work with what they have. Some tests are performance-only with horizontal motion, either in one direction or in the two orthogonal directions even when the shake table used is capable of moving vertically.7,8 In other cases, all three are used.9,10 Some shake tables are designed to be constrained to act as one larger shake table.
Shake table sizes vary considerably. Some are relatively small – 3 x 3 meters11 or 5.8 x 4.4 meters.12 The biggest in the world, E-Defense, measures 20 x 15 meters. Others fall in between, such as NIED’s Tsukuba Shaking Table, which is 14.5 x 15 meters. An extension frame can be added to make the platform larger.13
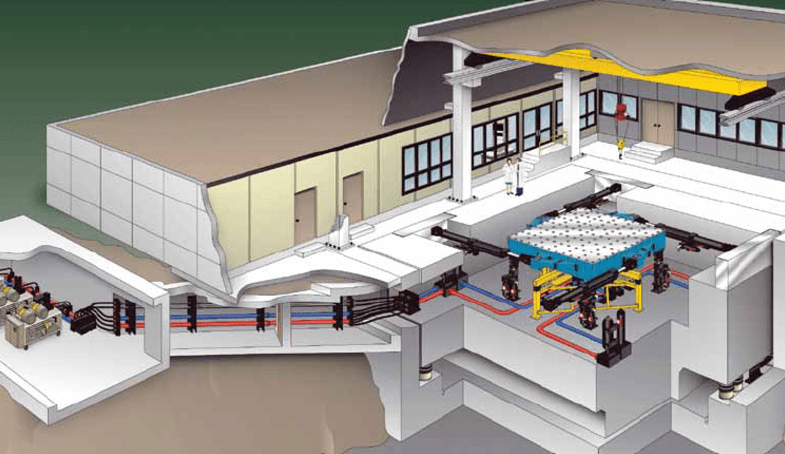
Figure 1a: Conceptual drawing of 6 degree of freedom seismic simulator facility
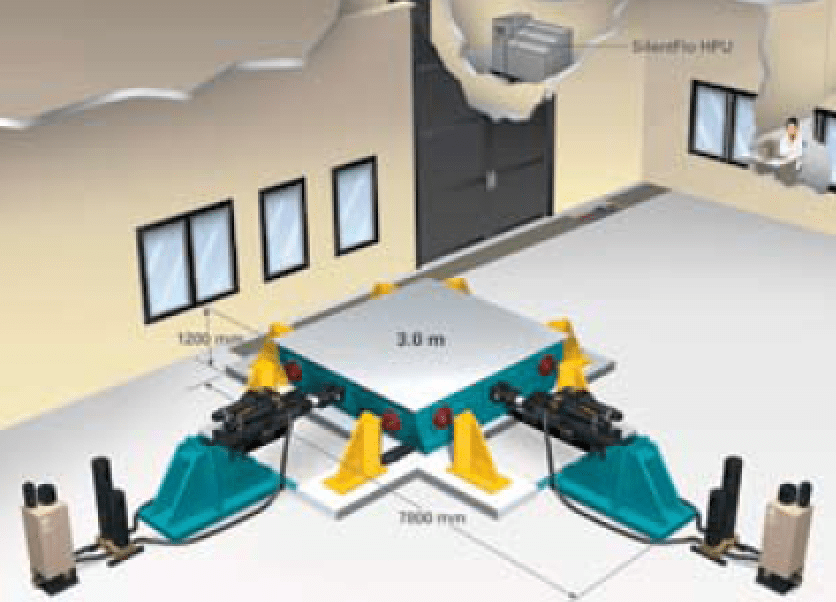
Figure 1b: Conceptual drawing of a bi-axial simulator
The input motion can vary depending on what the test is meant to evaluate. The movements can be sinusoidal wave, real input ground acceleration, ambient and forced vibration.14 “Real input ground acceleration” means that in an earthquake event, the movement was measured, and it is reproduced in the simulation.9,11,12,15,16 In some cases, loading history is used to expose the structure to a wide array of frequencies representing damage over time.13
Various types of sensors and hardware are used to measure the movement of the structure. Acceleration and displacement are often measured with strain-gage acceleration sensors. Triaxial geophones or voltage displacement transducers (LVDT’s) can be placed on the structure that convert movement into voltage that can be recorded. Video cameras are used for image processing for response displacements and retro-reflective targets can be placed to be picked up by the cameras for higher accuracy.13,16 Optical laser sensors can also be used.10 Sound and vibration can be measured with hardware such as the BBM data acquisition system.17 The data collected is used for qualitative and quantitative analysis. The data is often used as inputs for pseudo-static or pseudo-dynamic simulations (outlined in the next section) and are often used to confirm results.
The structures tested are composed of many materials. Materials include but are not limited to concrete walls cast in-situ,10 cross laminated timber panels,14 reinforced concrete,3,18 concrete hollow blocks,19 hollow bricks,11,16 and brick veneer.13 Sometimes the focus of the test can be anchoring, such as the anchoring of furniture to walls.9 Most tests do not include foundations and fix the superstructure directly to the shake table with bolts.6,13 Some exceptions to this general trend are shake table tests that have been performed on shallow foundations or retaining walls. In both cases the assemblies are placed in boxes of earth that has the desired soil mixture. The box is made of a steel frame with steel plates and sometimes glass panes are used on the sides to see the soil movement.17,20
Shake table tests are considered the norm in earthquake engineering research. Shake table tests are used to confirm theoretical and partial-experimental behavior.1 It is part of many building codes around the world and is often used to validate computer models and as a control group for trying out the other types of tests. Since it is expensive, the computer models are generated and tested once to find the accuracy of the model. If it accurately predicts the result of the shake table test, it is used for future calculations instead of repeating the shake table test.
Earthbags have undergone study and testing for compression strength, deformation characteristics, and general structural behavior,21-29 but only one shake table test has been performed on an earthbag assembly. The assembly was only 24 x 24 inches at 1:6 scale. However, the SolidWorks model gave accurate predictions of the behavior of earthbags. Three types of corner configurations were tested.30 Pakistan Straw Bale and Appropriate Building (PAKSBAB) along with the University of Navada tested a full-scale clay-plastered, thin, load-bearing straw bale house. Fishing net was used for reinforcement and the house was built on a gravel bag foundation that was encased in soil and cement and held in place on the shake table by formwork meant to simulate ground confinement. The house performed well, and damages were considered repairable. The gravel bags and straw bale were shown to dampen the movement.31
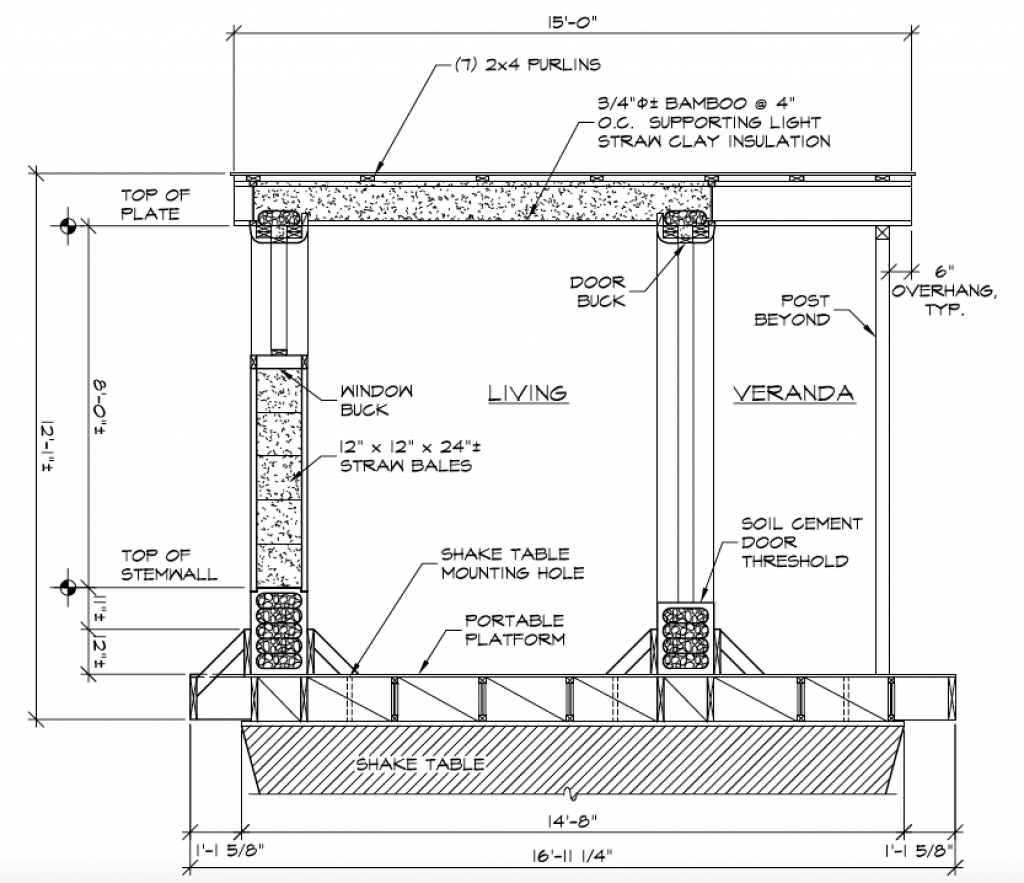
Figure 2: Section of PAKSBAB straw bale house tested at the University of Nevada
Pseudo-static and pseudo-dynamic testing
Pseudo-static testing is the representation (calculated by hand or in a computer program) of earthquake loads with “single constant unidirectional pseudo-static acceleration.” Pseudo-static testing uses the “statics” mathematical method that assumes the structure is in a state of static equilibrium. A pseudo-static coefficient must be selected and is the most important aspect of the calculations because it is a measure of the amplitude of inertial force.32 This method applies the force of a magnitude equal to the multiplication of the weight of the facility and the seismic coefficient. Earthquake loads are represented as a series of horizontal eigenvectors that are accumulated across the stories of a building and must be balanced using equivalent story stiffness and damping. Pseudo-static tends to agree with results evaluated by time history analysis.33
Pseudo-static tests have been used in the calculation of earth pressures behind retaining walls,34 slopes,35,36 earth dams,37 bricks,38,39 concrete,40 pile foundations,41 and concrete blocks.42
Pseudo-static is selected as testing method for large constructions where using a shake table is not an option such as earth dams or slopes. In the case of Li et al.,38 the research team had access to shake table, but it was too small for the structure they wanted to test. An advantage of pseudo-static is that it is a simple and straightforward analysis, but the disadvantage is that it is crude and doesn’t always accurately represent real affects. There are some dam projects that have failed during an earthquake even though they were shown to be resilient by the Pseudo-static method.32
Quasi-static testing is a physical test that can often accompany pseudo-static analysis by setting up actuators that apply predefined forces and then sensors that measure structural performance including rate of propagation of cracks, hierarchy of collapse, and levels of damage. It is usually performed on a partial construction or building assembly. The physical simulation involves setting up a hydraulic actuator that can push and pull the top of the structure and also simulate continuous vertical dead loads coming down on the structure.38 Horizontal cyclic loading is applied and then is increased step by step until the specimen cracks, losing its resistance to horizontal load.43 The disadvantage of this type of test is that it does not take into consideration the effects of inertia. An advantage is that it is possible to measure stress-deformation conditions near to failure which is not always possible with shake-table testing.14
Earthbag walls have been tested with quasi-static cyclic loading in order to determine the best way to construct them. They were low strength because of not using plaster and scaling them down. The conclusions of the test were tentative and explicitly said more testing is required to fully conclude the results.44
Figure 3: Quasi-static testing of earthbag corner.44
Pseudo-dynamic (PsD) testing is a more recently developed method that includes time in the calculation of applied earthquake loads and the effect of shear and primary waves. It incorporates computational modeling with the experimental testing that follows a feedback loop of the computer analyzing the resulting displacement and then using it to determine the response in the next time step. It is an experimental computer simulation that is creating new results and analyzing them as the program runs.45
The pseudo dynamic is reliable, informative and economical technique for small scale specimens.46 It can be used to analyze structures that are already in place and cannot be physically tested, such as internal steel frames.47 This also includes structures like retaining walls that can’t be analyzed any other way.48 It can be used to study full size structures as well, without the need of constructing the whole thing in a test rig.49 The pseudo-dynamic method has been found to be accurate when compared to codes and standards such as FEMA 27350 and GB50003-2001.38
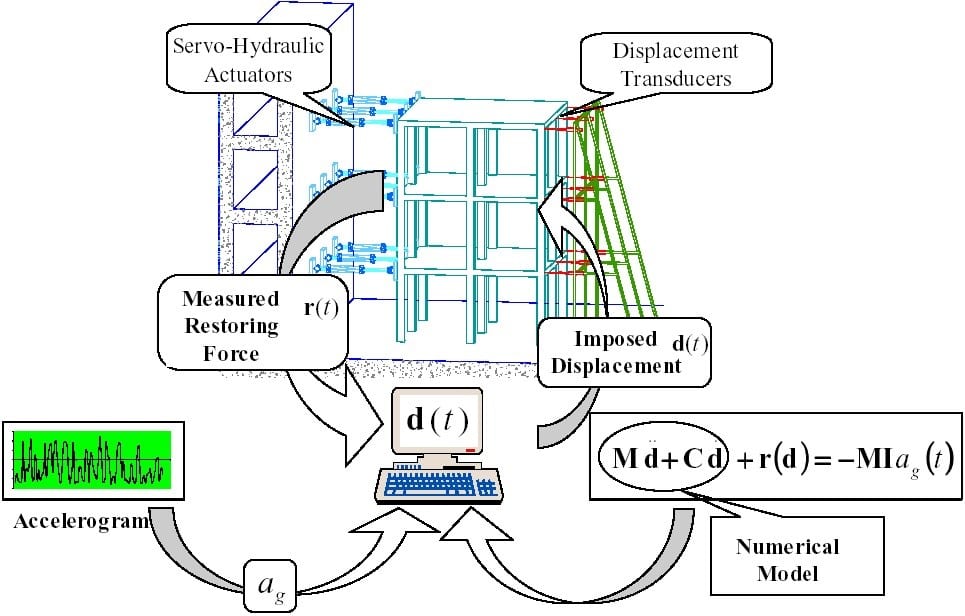
Figure 4: Diagram showing feedback loop of pseudo-dynamic simulation
Pseudo-static and pseudo-dynamic tests can be done by hand for simple structures such as a single retaining wall48 but can quickly become arduous for larger, complex structures necessitating the use of computers. Software used for pseudo-static and pseudo-dynamic testing include open-source, proprietary, and custom developed tools. Proprietary examples include ANSYS,3 Solidworks,30 and LS-DYNA.3 ANSYS and Solidworks are engineering simulation software whereas LS-DYNA is a multi-physics simulation software. Tensor Flow (TF) Multi is another multi-physics simulation software that is open source.31 An example of a custom software is FELISA//3M that was developed by the Institute of Earthquake Engineering and Engineering Seismology (IZIIS), Skopje.51
The programs modeling dynamic behavior use a method of analysis called Finite Element Method (FEM) that breaks down forces into a field of nodes that relate to one another.52 Wikipedia has a list of software packages that implement the finite element method for use by various applications. The calculations made from these programs often use inputs taken from shake table or quasi-static tests that represent the inertial forces, and the results are analyzed and compared.10 For granular or discontinuous structures or particles, a numerical simulating method called DEM or Extended Distinct Element Method (XDEM) are used. Researchers16 outline the use of a new simulating method based on XDEM applied to the simulation of earthquake forces on a brick house. In the computer simulation 2600 brick elements are calculated in relation to one another.
There has been no published pseudo-dynamic testing of earthbags.
Discussion
The shake table test and pseudo-static test give very close results.17,53 When compared with pseudo-dynamic, both gave similar results on earth pressure coefficients and expected start of failure.34 The main difference found between testing with a shake table and quasi-static is the stiffness. Pseudo-static is more conservative- it was also found that the value calculated with this method for collapse, when validated with a shake table test, was much lower than the actual collapse.10 Quasi-static provides information near to failure that is not possible with shake tests. Both are necessary to describe the behavior.14 In general, it is important to note that no matter what test is used, it might prove to be different to actual collapse modes.16
When earthbags are subjected to pressure, these tend to have plastic deformation, of even 50 mm.29 While loading tests like that one demonstrates the earthbags deform before collapsing, this means the behavior is different than that of masonry or concrete. When the latter are tested using the pseudo-static or pseudo-dynamic method, the bricks are modeled as single, rigid units, and the wall is pressured until it cracks. The ductility and the strength of the earthbags would make the modelling much more complex, since it doesn’t behave like rigid units. Shake table tests are often scaled down to make them more affordable; however, since there has only been one scaled test of a partial assembly, it is uncertain that scaled earthbags could accurately represent a full-size construction. Again, rigid elements like blocks and bricks have been scaled down, but earthbags could behave differently when loaded. Stouter44 indicates that only shake table testing would be able to reveal the vibration damping capabilities of earthbag walls. Based on this literature review it can be concluded that the best testing method for earthbags would be a full-scale shake table test. While this would be very expensive, a well-performed test could provide data to use in the development of more accurate computer simulation models, and be a one-time investment for future design.
References
- Ricci, I., Gasparini, G., Silvestri, S., Trombetti, T. (2012). Design of a shaking table test on a 3-storey building composed of cast-in-situ concrete walls. 15 WCEE Lisboa 2012.
- Duarte, R., Ritto-Correa, M., Vaz, C., and Campos-Costa, A. (1994). Shake table testing of structures. Earthquake Engineering, Tenth World Conference 1994 Balkema, Rotterdam.
- Dai, J., Yang, Y., and Weng, X. (2012). Shake Table Test for Large Indirect-Air-Cooling Tower Structure of Fire Power Plant – Part I. 15 WCEE Lisboa 2012.
- Sharma, R., Sachan, A., and Rai, D. (2012). Correlation between Pseudo-Dynamic and Shake Table Test on Steel Truss Moment Frames with Hysteretic Dampers. 15 WCEE Lisboa 2012.
- Singhal, V., and Rai, D. (2014). Suitability of Half-Scale Burnt Clay Bricks for Shake Table Tests on Masonry Walls. Journal of Materials in Civil Engineering. 26(4).
- Fujii, K., Ikeda, T. (2012). Shaking Table Test of Irregular Buildings under Horizontal Excitation Acting in an Arbitrary Direction. 15 WCEE Lisboa 2012.
- Nagashima, I., Hibino, H., Maseki, R., Narihara, H., Sato, E., Iiba, M., Kitamura, H., Yamano, Y., and Kashimoto, N. (2012). Multi-cyclic Dynamic Loading Experiment of Full-scale Devices for Seismic Isolation against Long-Period Earthquake Motions – Preliminary study. 15 WCEE Lisboa 2012.
- Branco, M., Guerreiro, L., Campos Costa, A., and Candeias, P. (2012). Shake Table Test of Structure Reinforced with Superelastic Dampers. 15 WCEE Lisboa 2012.
- Nagao, T., Kagano, H., and Hamaguchi, K. (2012). Full-Scale Shaking Table Test on Furnitures subjected to Long-Period Earthquake Motions. 15 WCEE Lisboa 2012.
- Ricci, I., Gasparini, G., Silvestri, S., Trombetti, T. (2012). Design of a shaking table test on a 3-storey building composed of cast-in-situ concrete walls. 15 WCEE Lisboa 2012.
- Petrone, C., Magliulo, G., Manfredi, G. (2014). Shake Table Tests for the Seismic Assessment of Hollow Brick Internal Partitions.
- Urban, M., and Stempniewski, L. (2012). Comparison of different earthquake strengthening methods for masonry buildings. 15 WCEE Lisboa 2012.
- Heath, D., Gad, E., Wilson, J. (2008). Shaking Table Test of Full-Scale Brick Veneer House.
- Hristovski, V., Stojmanovska, M., and Dujic, B. (2012). Full-Scale Shaking Table Tests of XLam Panel Systems – Correlation With Cyclic Quasi-Static Tests. 15 WCEE Lisboa 2012.
- Su, H., Wang, J., Jiang, X., and Tan, Y. (2014). Shaking table test on building in masonry structure with construction waste recycled brick. World Journal of Engineering. 11(4) 357-364.
- Hanazato, T., Minowa, C., Narafu, T., Imai, H., Ali, Q., Kobayashi, K., Ishiyama, Y., and Nakagawa, T. (2008). Shaking Table Test of Model House of Brick Masonry for Seismic Construction. The 14th World Conference on Earthquake Engineering.
- Yang, C., Zhang, J., Honglue, Q., Junwei, B., and Feicheng, L. 2015. Seismic Earth Pressures of Retaining Wall from Large Shaking Table Tests. Advances in Materials Science and Engineering.
- Tokui, N., Sakai, Y., Sanada, Y., Yamauchi, N., Nakano, Y., Suwada, H., and Fukuyama, H. (2004). Simplified Shaking Table Test Methodology Using Extremely Small Scaled Models. 13th World Conference on Earthquake Engineering.
- Imai, H., Inoue, H., Minowa, C., Lanuza, A., Penarubia, H., Narag, I., …Hanazato, T. (2012). Shaking Table Experiment for Philippine Full-Scale Concrete Hollow Blocks (CHB) Masonry Houses. 15 WCEE Lisboa 2012.
- Schneider, J.A., Moses, R.E.S., (2011). Linking cyclic stress and cyclic strain based methods of assessment of cyclic liquefaction triggering in sands. Geotechnique Letters 1:31-36.
- Croft, N. (2011). Structural Resistance of Earthbag Housing Subject to Horizontal Loading. Master Thesis, Bath University.
- Daigle, B. (2008). Earthbag housing: Structural behavior and applicability in Developing Countries. Master Thesis, Queen’s University.
- Dunbar, R. (2006). Prism Testing of Polypropylene Earthbags.
- Lohani, T., Matsushima, K., Aqil, U., Mohri, Y. and Tatsuoka, F. (2006). Evaluating the strength and deformation characteristics of a soil bag pile from full-scale laboratory tests. Geosynth. Int. 13:246–264.
- Stouter, P. (2010). Friction and Tensile Strength of Earthbag Components.
- Pelly, R. (2009). Plastic limit analysis of earthbag structures. Master Thesis, University of Bath.
- Tantono, S. (2008). The Mechanical Behavior of a Soilbag under Vertical Compression. Graz University of Technology: Graz, Austria.
- Vadgama, N. (2010). A Material and Structural Analysis of Earthbag Housing. Master Thesis, University of Bath.
- Ross, B., Willis, M., Datin, P., and Scott, R. (2013). Wind Load Test of Earthbag Wall. Buildings. 3(3):532-544.
- Malik, A. (2013). Structural Analysis of Earthbag Systems. Master Thesis, Thayer School of Engineering at Dartmouth.
- Champion, C. (2010). Seismic Response of Innovative Straw Bale Wall Systems and System Identification.
- Akhlaghi, T. and Nikkar, A. (2014). Evaluation of the Pseudostatic Analysis of Earth Dams Using FE Simulation and Observed Earthquake-Induced Deformations: Case Studies of Upper San Fernando and Kitayama Dams. Scientific World Journal.
- Saitoh, M., Ikegame, M., and Tanamura, S. (2004). A Quasistatic AnCalysis Method to Improve Collapse Mechanism Analyses of Multistory Buildings. 13th World Conference on Earthquake Engineering.
- Woodward, P., and Griffiths, D. (1996). Comparison of the pseudo-static and dynamic behavior of gravity retaining walls. Geotechnical and Geological Engineering. 14:269-290.
- Elahi, H., Poulos, H., Hajimollaali, H., and Elahi, A. (2018). Pseudostatic Seismic Response Analysis of a Pile Group in a Soil Slope. Geotechnical and Geological Engineering. 36(2):855-847.
- Presti, D., Marchett, D., Fontana, T. (2010). Pseudo-static vs. pseudo-dynamic slope stability analysis in seismic areas of the northern Apennines (Italy). Rivista Italiana di Geotecnica 2/2010.
- Akhlaghi, T. and Nikkar, A. (2014). Evaluation of the Pseudostatic Analysis of Earth Dams Using FE Simulation and Observed Earthquake-Induced Deformations: Case Studies of Upper San Fernando and Kitayama Dams. Scientific World Journal.
- Li, X., Gong, S., and Gu, X. (2004). Interaction and Seismic Capacity of Brick Wall and Supporting RC Frame. 13th International Brick and Block Masonry Conference Amsterdam.
- Wang, T., Zhou, M., and Ding, Y. (2017). A quasi-static test on a new type of masonry built of “I”-shaped closed-top hollow bricks. Australian Journal of Structural Engineering. 18(2):103-112.
- Twigden, K., Henry, R., and Ma, Q. (2014). Pseudo-static cyclic, snap back and shake table testing of PreWEC self-centering wall systems. Tenth US National Conference on Earthquake Engineering.
- Kumar, A., Choudhury, D., and Katzenbach, R. (2016). Effect of Earthquake on Combined Pile-Raft Foundation. International Journal of Geomechanics. 16(5).
- Bathurst, R., and Cai, Z. (1995). Pseudo-Static Seismic Analysis of Geosynthetic-Reinforced Segmental Retaining Walls. Geosynthetics International. 2(5):787-830.
- Russel, A., and Ingham, J. (2008). Flange Effects of an Unreinforced Masonry Wall Subjected to Pseudo-static In-plane Seismic Forces. The 14th World Conference on Earthquake Engineering.
- Stouter, P. 2016. Stronger Earthbag Corners: Test Results. Build Simple Inc.
- Jamal, H. (2017, Jul 07). Pseudo Dynamic (PsD) Tests – Non Linear Structural Dynamics Techniques. About Civil.
- Sharma, R., Sachan, A., and Rai, D. (2012). Correlation between Pseudo-Dynamic and Shake Table Test on Steel Truss Moment Frames with Hysteretic Dampers. 15 WCEE Lisboa 2012.
- Ozcelik, R., Binici, B., and Kurc, O. (2012). Seismic Retrofitting of Deficient RC Structures with Internal Steel Frames by Using Pseudo Dynamic Testing Procedure. 15 WCEE Lisboa 2012.
- Tafreshi, S. and Rahimi, M. (2012). A Simplified Pseudo-Dynamic Method of Reinforced Retaining-Wall Subjected to Seismic Loads. 15 WCEE Lisboa 2012.
- Negro, P., Bournas, D. and Molina, F. (2012). Seismic Testing of the SAFECAST Three-Storey Precast Building. 15 WCEE Lisboa 2012.
- Paquette, J., and Bruneau, M. 2004. Pseudo-dynamic Testing of Unreinforced Masonry Building with Flexible Diaphragm. 13th World Conference on Earthquake Engineering.
- Hristovski, V. and Garevski, M. (2013). Experimentally based analysis of Structures with FELISA/3M Software Package. SE-EEE Conference.
- Sattar, A. (2014, Mar 06). Simplest Explanation of Finite Element Analysis (FEA or FEM). Structural Engineering Tips.
- Oliva, M., Gavrilovic, P., and Clough, R. (1990). Seismic testing of large panel precast walls: Comparison of pseudostatic and shaking table tests. Earthquake Engineering & Structural Dynamics.
No Comments.